You all are welcome in this website. We shall know Thermodynamic Laws in Physical Chemistry. This chapter is very important with the purpose of transformation of energy. We shall study the conversion of energy into various forms with quantitative analysis. This topic is very interesting with the view of entrance and competitive exams to be held in coming session. We have done our best to solve all the topics along with zeroth, 1st, 2nd and 3rd law of thermodynamics.
Thermodynamic Laws in Physical Chemistry
The Zeroth law of thermodynamics
The credit for formulating this law goes to Ralph H. Fowler. This law states that if two individual bodies are in equilibrium with a third body that is separate, then the first two bodies are also in thermal equilibrium with each other.
This means that if the system A and system B have same temperature and the system C has also the same temperature with system A, then system B and system C will have also the same temperature. This state is said to be in thermal equilibrium.
First Law of Thermodynamics,
The first law of thermodynamics was given by Rudolf Clausius in 1850,
This law is also considered as the law of conservation of energy. This law states that energy can be neither created nor destroyed, but it can be changed from one form to another form without any loss.
Example- 1. In the operation of an electric fan, the electrical energy which is consumed is converted into mechanical work which moves the blade.
2. The decomposition of 1 mole of water into hydrogen and oxygen needs 285.8 KJ electrical energy. This energy must have been stored in hydrogen and oxygen since same amount of energy is released when 1 mole of water (liquid) is obtained from gaseous hydrogen and oxygen.
Second Law of Thermodynamics
Scottish physicist William Thomson, also known as Lord Kelvin, and German physicist Rudolf Clausius developed the second law of thermodynamics. This law states that all spontaneous processes proceed with a net increase of entropy, that means the total entropy change for all the spontaneous processes is positive.
Example- 1. Melting of ice proceeds with increase in entropy.
2. Decomposition of calcium carbonate on heating.
Third Law of Thermodynamics
This law was developed by the German chemist Walther Nernst between the years 1906
This law states that the entropy of all perfectly crystalline solids may be taken as zero at the absolute zero of temperature.
Example- When water vapour is continuously cooled down, it converts liquid and further into solid. Due to these changes, its entropy regularly decreases. Now that the temperature further cannot be lowered down than zero hence solid state is the last one and the entropy at this stage is zero.
Thermodynamic Terms in Physical Chemistry
To simplify the study the change in energy accompanying with chemical reactions, it is necessary to know certain thermodynamic terms. These are discussed below.
The System and the Surrounding
A system is the part of universe that is taken for the thermal consideration.
The remaining part of the universe besides system is called surrounding. That means system + surrounding = universe.
Boundaries.
The wall which separates the system from its surroundings is called boundary. It may be real or imaginary.
Types of the system
1. Open System. That system in which energy and matter both can exchange between the system and the surrounding is called open system. Example the presence of in an open beaker. Here the boundary is imaginary.
2. Closed System. That system in which energy can exchange but matter cannot exchange between the system and the surrounding is called closed system. Example tea in a kettle. Here the boundary is conducting.
3. Isolated System. That system through which neither energy nor matter can exchange between the system and the surrounding is called isolated system. Example tea in a thermos flask. Here the boundary is insulated.
The State of the System
That condition of a system which can describe the specific Volume, pressure, temperature of this system is called the state of this system.
State Variables or State Function
Those properties whose value depends on the final state and initial state of any system are called state Variables or state function. For example pressure, volume, temperature, amount of the substance, internal energy, enthalpy, entropy, free energy in a system.
Macroscopic properties
Those properties which are used to represent the large number of a substance are known as Macroscopic properties. For example Volume, pressure, amount temperature, density, melting point, boiling point etc.
Types of Macroscopic properties.
There are two types of Macroscopic properties. They are followings;
1. Extensive properties. Those properties which depend on the quantity of the matter contained in the system are called extensive properties. Example mass, volume, heat capacity, internal energy, enthalpy, entropy, Gibbs free energy etc.
2. Intensive properties. Those properties which depend upon the nature of the substance and are independent of the amount of the substance present in the system are called intensive properties. Ex:- temperature, pressure, refractive index, viscosity, density, surface tension, specific heat, freezing point, boiling point etc.
Thermodynamic equilibrium.
A system is said to be in thermodynamic equilibrium if its Macroscopic properties like pressure, temperature etc. Do not change with time.
Thermodynamic processes
When a system converts from initial state to final state by changing its energy. This change is called thermodynamic process. The following processes commonly met during the study of chemical thermodynamics.
A. Isothermal process. When the temperature of the system remains constant throughout the process. It is called isothermal process.
B. Adiabatic process. A thermodynamic process that is carried out in such a manner that no heat can flow from the system to the surrounding or vice versa is called adiabatic process. The system is completely insulated in this process.
C. Isochoric process. It is a process in which the volume of the system remains constant.
D. Isobaric process. The process during which the pressure of the system is kept constant.
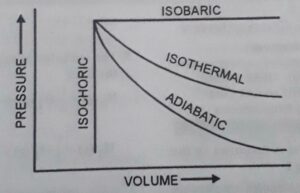
Reversible and Irreversible process
A process which is carried out so slow that all changes occurring in the direct process can be exactly reversed and the system remains almost in a state of equilibrium is called reversible process.
For reversible process,
Pexternal = Pinternal ± dp
A process that is carried out rapidly so that the successive steps of the direct process cannot be retraced and any change in the external conditions disturbs the equilibrium is called irreversible process.
Distinction between Reversible and Irreversible Process
Reversible Process | Irreversible Process |
1. This process is carried out infinitesimally slow. | 1. This process is carried out rapidly. |
2. At any stage during the process, equilibrium is not disturbed. | 2. Equilibrium may exist only after the completion of the process. |
3. It takes infinite time for completion. | 3. It takes finite time for completion. |
4. It is imaginary. | 4. It is real. |
5. In this process, work done is maximum. | 5. In this process, work done is minimum. |
Some Thermodynamic Quantities
Internal Energy
The energy that is already exists or stored within a substance in initial state is called internal energy. It is denoted by U or E. This energy is the sum of different types of energies associated with atoms and molecules present in a system or substance. All these energies cannot be measured exactly altogether and neither it is required to know the absolute value of internal energy of any substance or a system. Really we required internal energy change. Therefore we shall read internal energy change in details.
Internal energy change
The change in internal energy of a system when it goes from initial state to final state is called internal energy change. It is denoted by ΔU or ΔE. and ΔU = U2 − U1
ΔU = +ve if energy is absorbed
ΔU = – ve if energy is evolved
The internal energy of a system changes in the following conditions:
1. Heat passes in or out of the system
2. Work is done on or by the system
3. Matter enters or leave the system
Heat in Thermodynamic Laws
The mode of energy which exchange between system and surrounding due to difference in temperature is called heat is called heat. It is denoted by ‘q’.
Sign of ‘q’ :- when heat is given by the system to the surrounding, it is given negative sign. When heat is absorbed by the system, it is given positive sign.
Unit of heat or work:-
1 Litre atm = 101.3 J
1 calorie = 4.184 J
1 electron volt = 1.602 × 10-19 J
1 erg = 10-7 J
Work in Thermodynamic Laws
Work is said to have been done whenever the point at which force is applied is displaced in the direction of force. Two main types of work used in thermodynamics are followings
1. Electrical work. Here the force is termed as E.M.F. and displacement as quantity of electricity flowing through the circuit. Hence,
Electrical work done = E.M.F. × quantity of electricity = nFEocell.
2. Work of expansion. This type of work is involved in systems consisting of gases. This type of work is also known as pressure – volume work.
W = – Pext (V2 – V1) = – Pext × ΔV
For reversible expansion of an ideal gas. W = – 2.303nRTlogV2/V1.
Enthalpy in Thermodynamic Laws
The thermodynamic quantity U + PV is called the heat content or enthalpy of the system and it is represented by ‘H’.
H = U + PV.
Enthalpy Change. The amount of heat absorbed or evolved by the system at constant pressure is called enthalpy change. It is denoted by ΔH and ΔH = ΔU + PΔV
For a reaction: ΔH = ΔU + ΔngRT
Heat Capacity. The amount of heat required to raise the temperature of the system through 1oC is called heat capacity. It is denoted by ‘C’.
C = q/ΔT
Specific heat capacity. The amount of heat required to raise the temperature of 1 gram of the substance through 1oC is called specific heat capacity. It is denoted by Cm = q/mΔT.
Molar heat capacity. The amount of heat required to raise the temperature of 1 mole of the substance through 1oC is called molar heat capacity. It is denoted by ‘Cn‘ and Cn = q/nΔT
Types of molar heat capacities:
There are two types of molar heat capacities.
1. Heat capacity at constant volume (Cv)
2. Heat capacity at constant pressure (Cp)
Relationship between Cp and Cv.
1. Cp – Cv = R
2. Cp/Cv = γ
Where γ = 1.66 for monoatomic gases (He, Ar)
γ = 1.40 for diatomic gases (H2, O2, CO)
γ = 1.30 for triatomic gases (CO2, H2S)
Entropy in Thermodynamic Laws
This is the measurement of randomness or disorder of the system. It is denoted by ‘S’. This can be also not measured exactly hence we shall read entropy change.
Entropy change. The change in the value of entropy during a process is called entropy change. It is denoted by ΔS and ΔS = S2 – S1 = Σproducts – Σreactants.
As ΔS is related to q and T for a reversible process as: ΔS = qrev/T.
Sign of entropy change
1. If ΔStotal = +ve, the process is spontaneous.
2. If ΔStotal = – ve, the process is non- spontaneous.
3. If ΔStotal = 0, the process is in equilibrium.
Free energy change
That thermodynamic quantity of a system whose value decreases during a process is equal to the maximum possible useful work that can be obtained from the system. It is denoted by ‘ΔG’. And ΔG = ΔH – TΔS
Sign of ΔG:
1. If ΔG = – ve, the process is spontaneous
2. If ΔG = + ve, the process is non spontaneous.
3. If ΔG = 0, the process is in equilibrium.
Effect of sign of ΔH and ΔS and Temperature on the spontaneity of a process
Sign of ΔH | Sign of ΔS | Value of T | Spontaneity |
---|---|---|---|
– | + | Any | Spontaneous |
+ | – | Any | Non- spontaneous |
– | – | Low | Spontaneous |
– | – | High | Non- spontaneous |
+ | + | High | spontaneous |
+ | + | Low | Non- spontaneous |
Thermochemistry
The branch of chemistry which deals with the heat changes during chemical reactions is called thermochemistry.
Types of chemical reactions:
There are two types of chemical reactions according to heat exchange in a reaction.
1. Exothermic reaction. Those reactions in which evolution of heat take place is called exothermic reaction. For example
C(s) + O2(g) → CO2(g) + 393.5 KJ
ΔH is negative for exothermic reactions.
2. Endothermic reaction. Those reactions in which heat is absorbed are called endothermic reactions. For example.
N2(g) + O2(g) →2NO(g) – 180.7 KJ.
ΔH is positive for endothermic reactions.
Heat of reaction or enthalpy of reaction
The amount of heat released or absorbed in a chemical reaction when a number of moles of the reactants have completely reacted is called heat of reaction or enthalpy of reaction. It is denoted by ΔrH. For example.
CH4(g) + 2O2(g) → CO2(g) + 2H2O (l), ΔrH = – 890.4 KJ mol-1.
Standard enthalpy of reaction
The enthalpy of a reaction when all the reactants and products are in the purest and most stable form at 1bar pressure and 298K temperature is called standard enthalpy of reaction. It is denoted by ΔorH.
Different types of enthalpies of reaction.
1. Enthalpy of combustion. The enthalpy change during a process in which 1 mole of substance is completely burnt or oxidized in oxygen is called enthalpy of combustion. It is denoted by ΔcH. For example.
CH4(g) + 2O2(g) → CO2(g) + 2H2O (l), ΔcH = – 89Δries or joules produced when 1 gram of food or fuel is completely burnt is called calorific value of this food or fuel. For example the calorific value of Glucose = 2840/180 = 15.78 KJ g-1.
2. Enthalpy of formation.
The amount of heat change when 1 mole of the substance is formed from its elements under given conditions of temperature and pressure is called enthalpy of formation. It is usually represented by ΔfH. Enthalpy of formation for any substance in the standard state is called standard enthalpy of formation. It is denoted by ΔfHo. For example
C(s) + O2(g) → CO2(g) + 393.5 KJ
Here ΔfHo = – 393.5 KJ mol-1.
Importance of standard enthalpy of formation.
1. ΔrHo = ΣΔfHo(products) – ΣΔfHo(reactants)
2. For elementary substances in the standard state, the standard enthalpy of formation is taken as zero. For example
ΔfHo for H2(g) = 0, ΔfHo for Br2(l) = 0, ΔfHo for Cl2(g) = 0.
3. Enthalpy of neutralization
The enthalpy change when 1g-eq of an acid is neutralized by base or vice – versa is called heat of neutralization. It is denoted by ΔneutH.
NaOH + HCl → NaCl + H2O
Here ΔneutH = – 57.1 KJ mol-1.
Note.1. In case of strong acid and base, ΔneutH = – 57.1 KJ mol-1.
2. In case of a weak acid or weak base or both are weak, ΔneutH < – 57.1 KJ mol-1.
4. Enthalpy of solution
The amount of heat evolved or absorbed when 1 mole of the substance is dissolved in a specified amount of the solvent is called enthalpy of solution. It is represented by ΔsolH. For example-
KCl(s) + aq → KCl (aq), ΔsolH = + 18.6 kJ mol-1
ΔsolH = ΔlatticeH + ΔhydH,
Note:- Greater is the lattice enthalpy of an ionic compound, less is its solubility in water.
5. Enthalpy of atomization
The amount of heat or enthalpy change during a process in which 1 mole of a given substance dissociates into gaseous atom is called enthalpy of atomization. it is represented by ΔaHo. For example,
H2(g) → 2H(g) ΔaHo = 435.0 kJmol-1
6. Bond Enthalpy or Bond Energy
The amount of energy required to dissociate one mole of bonds present between the atoms in the gaseous molecules is called bond enthalpy. It is represented by ΔbH.
Note: 1. For diatomic molecules like H2, O2, N2, HCl, HF etc, the bond energies are equal to their dissociation energies.
2. For polyatomic molecules, the bond enthalpy is the average of the sum of the bond enthalpy of each bond.
Formula for bond enthalpy. ΔrH = ΣB.E. (reactants) – ΣB.E. (products)
Hess’s Law of Constant Heat Summation
According to this law- The total amount of heat evolved or absorbed in a reaction is the same whether the reaction takes place in one step or in a number of steps. For example
When 1mole of carbon burns to form carbon monoxide first which then burns to form carbon dioxide, The heat evolved in these two steps are – 110.5 kJmol-1 and – 283.0 kJmol-1 respectively . These are the sum when 1 mole of carbon (graphite) burns to form carbon dioxide directly in one step, 393.5 kJmol-1 of heat is produced.